This article is adapted from a study that first appeared in the International Journal of Sports Science & Coaching, Vol.11(3) 422-435, 2016. In additional to following the development of the American junior record holder, it almost serves as a guide to advanced technique and training for the hammer and the benefits sport science brings to coaching the event
By Lawrence W. Judge, Ball State University; Mike Judge, Throw1Deep Sports; David Bellar, University of Louisiana at Lafayette; Iain Hunter, Brigham Young University; Donald L. Hoover, Western Kentucky University, & Rhonda Broome, Throw1Deep Sports.
ABSTRACT
The purpose of this case study was to describe the integration of sport science and coaching that helped to produce an American junior record (AJR) of 68.12m (223’6”) in the women’s hammer throw in 2012. Two digital video cameras (Canon Elura 60) running at 60 Hz were placed to view Shelby Ashe’s best throws at the 2010 USATF Nationals (61.77m—202’8”), 2011 USATF Junior Nationals (58.34m—191’5”), and 2012 USATF Junior Nationals (68.12m AJR). Following each competition the coach collaborated with the sport scientist and chair of USATF coach’s education to review and evaluate each of the throws. The record setting throw by Shelby Ashe was 1.49 seconds in duration from right foot lift-off and produced a release velocity of 26.8 m/s. During the throw, Ashe spent 50.35 percent of the duration of the throw in double support. Improvements in technique focused on strategies designed to increase velocity of the head of the hammer.
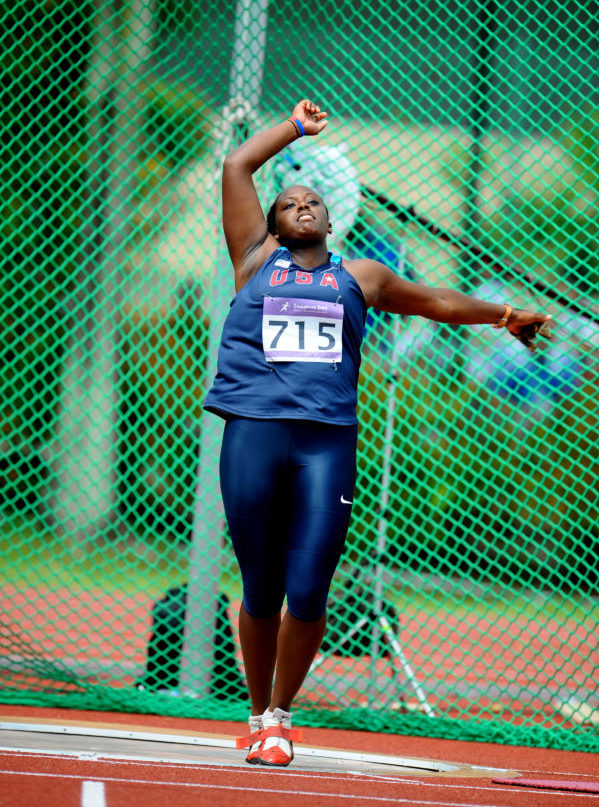
INTRODUCTION
The hammer throw is an explosive event in which the competitive athlete performs between three and four rotations within a 2.135 meter ring and releases an implement with a mass of 7.26 kg and length of (121.5 cm) for male competitors, or a mass of 4 kg and length of (119.5 cm) for female competitors. The difference in implement weight does factor into the amount of force needed to overcome inertia and to balance against the centrifugal forces. The men have greater differences in time for each individual turn as they progress through the throw [1].
Due to larger amounts of inertia, men begin slowly and increase their speed at a greater rate than women early in the throw [1-3]. Men turn more rapidly during the last two turns than women [4].
All athletes involved in this event must generate as much velocity with the implement as possible, while maintaining balance and coordination through the sequence of turns [5]. Although the trunk and upper-body musculature are used in a throw, the legs provide the larger muscle groups that initiate movement of the hammer. The initiation of the large muscles of the legs helps create tension along the wire (pulling the wire taut). This action is followed by isometric actions of the trunk and upper-body musculature to allow for a larger turn radius.
The thrower will then assume an approximate quarter-squat position when performing 3-4 progressively accelerating turns to reach optimal rotational velocity prior to triple extension and subsequent release of the hammer [6,7]. The ball speed of top male and female throwers at release has been measured between 20 to 27 m/sec [8]. The athlete needs to develop a strong but flexible vertebral column and hip joint to enable a wide range of movements and body torque.
In the United States, high school athletes have limited exposure to the hammer throwing event. For that reason, there is nearly a complete reliance on the collegiate system to develop international-level competitors in the hammer throw. In the 1940’s, as America’s population began to rapidly grow, the interscholastic associations of 23 state and 93 private secondary schools discontinued hammer throwing [7]. Only the smallest state, Rhode Island, continued to support high school hammer throwing, and it has to this day. Emphasis on football, baseball, and later basketball and hockey grew in the United States. These sports were subsidized by colleges and professional franchises [7], and reigned supreme among American youth. In contrast to the United States following the recovery from World War II, Eastern and Western European athletics federations began training pre-pubescent and adolescent hammer throwers, encouraging and praising achievements without the competition of marketing and the allure of college and professional sports that American youth experienced [7].
However, as the National Collegiate Athletic Association (NCAA) made the women’s hammer throw and weight throw (indoors) official events in 1996, interest in the hammer throw once again developed in the United States. Universities and colleges in the United States invested resources into the event (i.e. new coaches, scholarship money for athletes, and new facilities). Additional opportunities to earn scholarship money were available for female athletes. Presently, you will find pockets of hammer throwing clubs developing in the states of California, Georgia, New York, and Washington [7].
The women’s hammer movement in the United States started impressively in the 2000 Summer Olympics in Sydney, where Dawn Ellerbe finished in 7th place in the women’s hammer throw competition with a distance of 66.80 meters (219’2”), and USA teammate Amy Palmer finished 8th [7]. Ellerbe was the first American female to throw over 70 meters and established the American record of 70.46m (231’2”)on her way to winning the gold medal at the 1999 Pan American Games [7].
In recent years, the hammer throw has evolved into a highly technical model, thus the need for an understanding and applying scientific principles to the event. Understanding the mechanics of the event allows the coach to more readily identify technical issues that may be an impediment to performance.
The mechanics of the hammer throw are complex, as the movement involves rotations of the hammer in varying planes, coupled with the translation and rotation of the thrower across the throwing circle [10].By understanding and applying scientific principles, the throws coach will be able to more accurately identify needed technical modifications and devise training stimuli in an effort to better accommodate the athlete, resulting in improved performances [11].
Accurate and scientific measurements of contributing motions, particular to an action, can be determined through a kinematic analysis of slow-motion cinematography. Anthropometric differences will affect the time for each individual turn, distance thrown, and overall execution of the complete throw [2]. In the hammer throw, the ability to maintain ground contact with both feet for as long as possible will enhance the acceleration of the ball [1,8,12]. The ball will have a longer acceleration path allowing for an increase in velocity to occur. Measuring variables like time spent in single support (SS) verses double support (DS) and shoulder/hip separation in the initiation of DS can assist the coach.
Throughout the hammer throw, the speed of the hammer fluctuates, primarily as a result of the tangential component of the cable force (tangential force) fluctuating between positive and negative [13]. Previous literature suggests that the hammer (men’s or women’s) can only be accelerated in the DS phase [2,5]; as it is not possible for the thrower to actively influence the velocity in the SS phase [14]. However, it has been suggested that throwers may impact the speed of the hammer during the SS phase by increasing the vertical velocity of the hammer [15]. The majority of literature focuses on strategies that may be used by throwers to actively increase the speed of the hammer within each turn, specifically during the DS phase with little focus being put towards how throwers could reduce the size of losses in speed in the subsequent SS phase. Ideally, the losses in hammer speed that occur during the SS phase can be minimized [16].
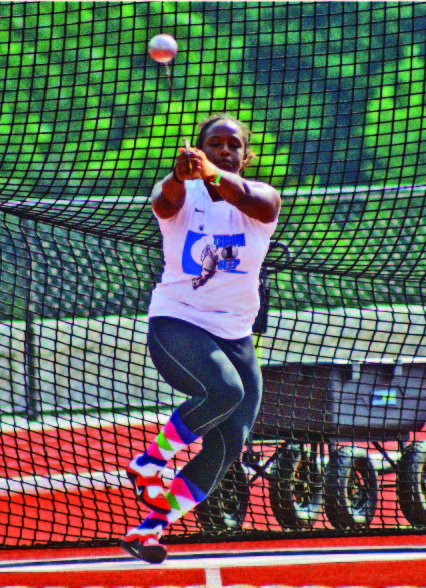
The three determining factors of throw success are the velocity, height, and angle at release [11,17,18]. Of these, the most important factor is velocity at release [11]. Evaluation of biomechanical research can significantly impact the performance of athletes in the hammer throw when properly utilized and understood by coaches. Evaluation of this type of data may result from careful analysis of video segments of individual athletes.
The variety of conclusions that may result from careful analysis of a segment of video on an individual athlete depends upon a number of factors related to the nature of video. Those factors include the way in which the video was recorded and the skills of the videographer and researcher. Additionally, atypical throws by an athlete will complicate accurate analysis of data, making it necessary to record several throws in order to identify commonalities in the typical throwing motion of any given athlete.
The coach and researcher (sport scientist) must work together to identify errors and improve technique in the hammer throw. Beyond developing distinct, event-specific motor abilities, factors like training status, training age, chronological age, genetics (e.g., fiber-type composition), anthropometrics, gender, relative strength, and absolute strength have an influence on training emphasis.
The purpose of this case study was to describe the USATF Coaches Education hammer project in which the cooperation between sport science and coaching helped to produce an American junior record of 68.12 meters thrown by Shelby Ashe in the women’s hammer in 2012. This research conducted a biomechanical analysis of American hammer thrower Shelby Ashe (Figure 1) at the 2010, 2011 and 2012 United States Track and Field Junior Nationals. The methodology and procedures will be presented as a guide for coaches and sport science researchers desiring to analyze the technique of athletes through a process that utilizes high-speed film and training data analysis.
METHODS
Subject
The authors’ Institutional Review Board approved this project. The participant included one high school athlete, from North Central Georgia, who had never thrown the hammer at the initiation of this project started in 2010. At the start of the project, the athlete was of normal size and weight for a competitive high school track and field thrower, and had been cleared to participate in athletics via the local sports medicine staff. After giving consent, the coach was asked to report via a datasheet the age, height and weight of the athlete, throwing volume, as well as her season bests in the hammer throw and weight throw event and weight room 1RM for the bench press, power clean and squat exercises. In total, the datasheet consisted of eight items.
Following the coach’s report, the data for the athlete was entered into a spreadsheet program, and the data report sheet was destroyed. The athlete was new to the hammer throw, and years of resistance training were 0.50 years at the start of the project in 2010. In 2012, the subject was measured at a height of 173cm (5’8”), weight of 83 kg (183 lbs), and body fat of 15.9% (see Table 1 for anthropometric data).
Procedures
Two digital video cameras (Canon Elura 60) running at 60 Hz were placed to view Shelby Ashe’s (Tables 1 and 2) best throws at the 2010 USATF Nationals (61.77m), 2011 USATF Junior Nationals (58.34m), and 2012 USATF Junior Nationals (68.12m American Junior Record). One camera was placed perpendicular to the throwing direction in an effort to obtain the release angle. Release velocity was calculated using the explanation by Hunter [19]. The other camera was placed behind the athlete to determine the length of time in single and double support phases [during each turn of the hammer throw, the period of time spent with both feet on the ground is known as double support (DS), and the period of time spent with only one foot on the ground is known as single support (SS)].
The Dartfish ProSuite 4.0 was used to measure release angle, height, and support phase duration. Following each of the competitions in 2010 and 2011, the coach collaborated with the sport scientist and chair of USATF coach’s education in order to review and evaluate each of the throws. Based upon an evaluation of recorded data, a detailed biomechanical analysis of the throws was created.
Training Program. The overall scheme of Ashe’s strength and conditioning (SC) plan utilized a linear periodized format that included short, intermediate, and long-term goals involving planned distributions of workloads [20]. The exercises during this initial training period incorporated the combination of strength, power, and reactive strength exercises (plyometrics), which have demonstrated the ability to greatly increase vertical jump height than if either one was performed in isolation [21]. Furthermore, training at varying intensity levels in different phases may be more effective for increasing strength than training at a continuously high intensity [22]. It is critical that the SC plan progress from basic hypertrophy and strength in the General Preparation Phase (GPP) to explosive strength in the Competition Phase (CP).
The development of the S&C plan began with a GPP and transition to a Specific Preparation Phase (SPP), then finally transition into a CP. The GPP is not 100% specific to the athlete’s goal, but is well suited for a junior athlete. However, as Bompa and Haff [23] stated, “The ultimate goal of (general physical training) is to improve the athlete’s working capacity and maximize physiological adaptations to prepare the athlete for future workloads.” During this phase, the bio-motor attributes to be developed are muscular strength, speed, flexibility, coordination, and event-specific endurance [23, 24].
Cleans, snatches, pulls, squats, jumps, and sprints comprised the GPP focusing on technique prior to advancing the intensity or more complex movements (e.g. depth jumps with sprint).
The mesocycle sequencing was based on the linear model used for hammer throwers established by Judge et al. [25], which progresses through methods in this order; hypertrophy, strength building, neural activation, and finally speed-strength methods. This was repeated three times annually for a total of four rotations. For example, the mesocycle sequencing included the first mesocycle length of up to 8 weeks if the athlete requires muscle mass (mid-Aug.—early Sept.). The second mesocycle (late Sept. – early Oct.) emphasizes basic strength with an emphasis on improving the squat [26, 27]. The third mesocycle (late Oct.—early Nov.) emphasized strength/power using 3-4 weeks of neural activation methods with the emphasis on Olympic lifts and Olympic lifting derivatives and plyometrics [28]. The fourth mesocycle (late Nov.—early Dec.) emphasized explosive power and speed development using time-controlled speed-strength methods. The sequence was repeated following a regeneration period (December holiday) [25].
The degree of loading for each exercise was described as a percentage of the one repetition maximum (1 RM). For example a loading of 80–90% of 1RM (2–5 reps) was utilized for lifts and their derivatives that encompass the sport of weightlifting. For maximum peak power development, 30–40% of 1 RM was utilized on exercises like the jump squat and narrow grip snatch during the competitive phase. The number of sets varied for each exercise and repetition range. For the lower repetition ranges (6 or less) about four to five sets per exercise were performed. For the higher repetition ranges (8 to 10 repetitions), two to three sets per exercise were performed. Rest between lower repetition range sets was high (3 to 5 minutes) and rest following high repetition sets was lower (60 to 90 seconds).
A two-week transition or active recovery phase was initiated during the month of December during which time testing was conducted to evaluate the thrower’s adaptations from the training plan and also a video analysis was completed of any skill instruction that may need further improvements [25]. During the active recovery phase, incorporating easy sprints or strides (e.g. distances < 40 m, with effort <100%), body weight strengthening exercises or calisthenics, and light medicine ball throws (e.g. < 4 kg) in a circuit format maintains a structured plan for the athlete while decreasing the possibility of injury and maintaining the progression achieved during the GPP. The structure of skill practices, a balanced training plan, and reducing external physical stressors was designed to decrease the chance of injury with the thrower’s increased participation in competitive events during the season [25].
A physical therapist was consulted to help design prophylactic exercises to integrate into the training program. In addition to the weight lifting exercises, throws, sprint drills, and jumps, the workout contained sport-specific release movements that force core stabilization of high velocity activities. Sport-specific exercises that mirror sport-specific release parameters are an effective way to develop event-specific strength. Heavy weights (20 or 25 pounds) are used for power and lighter weights for speed. These exercises were designed to emulate key sport-specific release positions [25].
While specialized training provides great benefits, any enhancement of power can be severely restricted if general strength parameters, mobility, and posture are not also addressed. Similarly, not only may an athlete’s power generation abilities be limited, he or she is often at greater risk of injury if, for example, musculoskeletal imbalances that contribute to abnormal static and dynamic and postures are not addressed prior to initiating a periodization plan. Paralympic throwers in particular are susceptible to musculoskeletal imbalances of this type, in part due to the chronic effects of asymmetrical biomechanical demands placed upon the body during throwing motions.
The literature has many examples of throwing athletes who sustain injury to musculoskeletal structures, nervous tissue, or both due to repetitive microtrauma to these areas [29, 30, 31, 32, 33] Thus, a physical therapist was consulted to help design prophylactic exercises to integrate into the training program. The physical therapist completed an initial evaluation on the athlete, and he identified musculoskeletal imbalances and prescribed therapeutic exercises to address these limitations [34, 35, 36, 37, 38]. The throwing coach 1) integrated these prescribed activities into the athlete’s annual training program with the physical therapist’s input, and 2) had the physical therapist re-examine the athlete a couple times throughout the year and modify these prophylactic exercises as needed so as to foster her power development in an injury-free manner.
Throwing Sessions. Each training session began with a warm-up protocol that included 10-15 minutes of general warm-up activities (skipping, dynamic mobility, etc.) consisting of short runs (>30 meters) of increasing intensity, dynamic stretching, body weight exercises, and medicine ball drills. The general warm-up was designed to increase muscle temperature and increase blood flow.
The specific warm-up period was similarly five to 10 minutes in length and consisted of sport-specific movements designed to prepare the athlete for the demands of the sport. The specific warm-up began with utilization of a car tire to turn in each direction. The athlete completed 20 turns (5 sets of 4 turns) right-handed and 20 turns (5 sets of 4 turns) left-handed. The purpose of this activity was to warm the leg and hip muscles in preparation for the training session. This drill was typically followed by six wind and turn drills with release. Next, the athlete completed 6 sets of right hand only throws followed by 12 full throws utilizing the three-turn technique. Following the completion of the specific warm-up throwing sessions, utilizing varied weight and length implements was initiated. Typically a morning (AM) throwing session consisted of 12 to 16 throws and an afternoon (PM) throwing session consisted of 25 to 40 throws. The athlete finished each throwing session with special strength work (i.e. 10 one-arm PUD releases) designed to help the athlete improve the release.
The weight practice for indoor season incorporated either a 30lb weight or an 8K short hammer. The athlete threw the medium length implement in order to try to incorporate and maintain hammer timing and techniques as well and to prepare for the indoor weight throw event.
Following the 2011 season, the volume of throws was drastically increased due to the relatively young training age of the athlete and to make up for the lack of experience with the hammer. With the World Junior championships quickly approaching, increasing the demands of training was determined as the best way for Shelby Ashe to secure a position on the U.S. Junior national team.
The number of total throws increased from 2720 in 2010 to 3376 in 2011 (Table 3). Keep in mind that these numbers included every activity that was completed with a delivery. This consisted of full throws, drills with a release, and one- and two-turn throws with a release. Of the 2720 total throws in 2010, 1170 were performed with the weight, and 1550 were performed with the hammer. In 2011, 1404 throws were performed with the weight, and 1972 throws were performed with the hammer. Throws were completed with hammers ranging from 3.5 kg to 6 kg. Throws were completed in the weight with implements ranging from 9 kg to 14.5 kg. The majority of the weight throws were performed in the fall and winter months, with the majority of the hammer throws being performed in the spring and summer months.
During the preparation and pre-competitive phase, three throwing workouts were performed daily. This was reduced to two workouts per day during the competitive phase. Two training days were generally followed by one recovery day. In 2012, 1404 throws were performed with the weight, and 2284 throws were performed with the hammer equaling a total of 4070 (see Table 4). During the preparation and pre-competitive phase, two throwing workouts were performed daily. This remained the same during the competitive phase. Two training days were generally followed by one recovery day. Throws were completed with hammers ranging from 3.5 kg to 7.26 kg. Throws were completed in the weight with implements ranging from 9 kg to 16 kg. During 2012, the emphasis was on improving maximum strength in the lower body.
Parameter Selection. A technical model of the elite hammer throw was used for a technical intervention between 2010 and 2012. This technical model was developed from the findings of previous research [25]. The goal of this prior research was to determine the most critical variables for success in elite women’s hammer throwing. Variables of interest were based upon previous work [25, 39, 40], which demonstrated relationships between maximum strength in the squat, power clean, and track & field throwing event performance and also included known differences by gender. Variables of interest were analyzed for relationships with season’s best performance via partial correlations. Subsequently, semi-partial correlations were used to assess the strength of the relationships among the 1RM assessments and the season’s best throw.
Although release velocity is clearly a significant indicator for performance, it was excluded from the variables because it does not reveal any applicable information to an athlete because most athletes are presumably trying to throw as hard and fast as possible during a competition. Likewise, because previous research [31] has indicated that release velocity explains so much of the variance of a throw by itself (90+%) it was concluded that it could mask the importance of other variables that may be more applicable to beneficially effecting a technical intervention.
Data Analysis
The method employed in this study to breakdown the throw into phases by predetermined events allowed for comparison of this research with previous research based on hammer throw performance. This method has been used previously to examine the hammer throw [25]. A modern statistical software package was used to perform the analysis (SPSS ver 20.0) and statistical significance was set a priori at alpha<0.05.
RESULTS
Release angles in this case study were consistent among all throws. However, a relationship between release velocity and throwing distance is noted as release velocity increased with throw distance (Table 5). Ashe’s American junior record throw produced a release velocity of 26.8 m/s. No trends were observed in support phase times (Tables 6-8). However, the 2010 throw showed a relatively longer total time in turns two and three. The record-setting throw by Ashe in 2012 was 1.49 seconds in duration from right foot lift-off. During the throw, Ashe spent 50.35 percent of the duration of the throw in double support.
From 2010 until the 2012 season, Ashe’s back squat and power clean personal bests improved by 22.6% and 23.5% respectively (see Table 1). Whereas increases in personal bests in weightlifting movements that require higher bar speed, such as the snatch and explosive movements such as an overhead back shot throw increased by 15.8 and 7.9%. The subject’s average (mean) throw increased each season. In 2010, the number of competitions was 14 with a mean distance of 57.94 + 4.98m. In 2011, the number of competitions was nine with mean distance of 61.54 + 2.27m. In 2012, the number of competitions was 10 with a mean distance of 63.20 + 2.86m.
DISCUSSION
An important characteristic of effective coaching is the ability to recognize the critical components of athletic performance. Highly effective coaches utilize critical components of performance in order to create training protocols designed to help the athlete reach maximum performance. Shelby Ashe demonstrated various biomechanical characteristics of the hammer throw which distinguished her from other elite junior hammer throwers. The results of the present study indicate this success was due to an efficient technical pattern and a balanced approach to training. One of the primary findings of this case study was that from the 2010 until the 2012 season, Ashe’s back squat and power clean 1RM (personal bests) improved by 22.6% and 23.5% respectively. Number of throws per year and number of years, back squat and hammer throwing technique were significant predictors of hammer throw distance in a study by Judge, Bellar, McAtee and Judge [39]. The increases in throwing volume and 1RM’s in the squat and power clean from 2010 until 2012 in the present case study are supported by the investigation by Judge et al. Trends in the data in the present case study were comparable to a similar case study conducted by Judge et al. [25].
It is important for coaches to be able to identify and sequence the training effects that contribute to sport form in the hammer throw. Maximum strength in the squat exercise was a significant predictor of the personal best in a closely related event (the indoor weight throw) for the subjects in a study by Judge, Bellar, Turk, Judge, Gilreath, and Smith [40]. The results of the present case study investigation agree with the information that was found in related work on the hammer throw [25, 39] and the shot put [42-44]. Results of an investigation by Judge and Bellar [42] reported that strength in the power clean was strongly related to the distance achieved in the shot put event. Reis and Ferreira [44] evaluated the validity of several strength and power tests to predict performance in the shot put. The study provided mixed results as some tests of power (such as a variety of jumping tests) did not correlate with performance where throwing tests (power) and weight lifting tests (1 RM strength) showed a significant association with performance. Strength is a necessary component in the hammer throw because it enables the athlete to hold the technical positions while moving at high velocities [25]. Counteracting the effective weight of the implement at the low point of the elliptical orbit and imparting torque on the implement to further its rotation are both technical skills requiring the same type of core strength as in the squat [40]. Therefore, the large increases in the 1RM of a structural multi-joint exercise like the squat lift by Ashe for increasing strength and hypertrophy of the core and back extensors was not surprising [40].
The Entry
The technical components of Ashe’s entry presented a significant challenge to the training program. In her second year throwing, 2011, Shelby was not very comfortable with her winds and entry. This aspect created a significant challenge, as the winds are one of the most important technical elements of the hammer throw. The winds, especially in Ashe’s three-turn technical model, were responsible for the throwing rhythm and tempo, the position of the lowest point in the rotational trajectory of the hammer, the movement of the head of the hammer into an optimal position and the placement of the athlete’s body into the appropriate position for the turns. Execution of the preliminary swings (winds) required stability [25]. A methodical development of this important technical element was essential prior to beginning the throwing action as a whole. Ashe demonstrated difficultly with maintaining relaxed arms on the winds, and she appeared to be off-balance. Her winds impacted her balance on the start, which tended to decrease her turning speed on turns two and three.
One of the differences from 2011 to 2012 was the consistency of the start. This consistency was developed as a result of the adjustments made to widen Shelby’s initial starting position. In order to create maximal rotational momentum, the suggested placement of the feet is wide (70cm to 80cm) [2, 25]. Minute changes (as little as 2 cm) to this position influenced the subsequent structure of the throw [25]. Ashe began throwing in 2010 with a narrow base of 65cm but maintained balance and stability in her starting position through gradually using a wider base (80cm). Most three-turn throwers that were studied as a technical model obtained a steeper orbit earlier in the throw. The objective on the start for Ashe was to achieve a relatively flat orbit on the entry turn. This flat orbit allowed Ashe to be on balance as she strikes the ball on the final wind. Because of the adjustment, Ashe was able to maintain a greater than 40-degree release angle while remaining controlled by making a more gradual progression to increase the orbit.
The mechanics of the throw were profoundly impacted by the position of the low point following the winds. In 2010, Ashe set up her low point a little right of center. Throwers are typically better able to accelerate the implement in the first turn by positioning of the low point of the trajectory a little to the right of the center line. However, doing so may create a challenge to produce a wide hammer arc movement to the left.
As she became more technically proficient in 2011 and 2012, the low point was set up at zero degrees (exactly on the center line). It was believed that athletes with a high level of speed capacities and in complete control of the technique have no need to “advance” the low trajectory point by 40 to 45° to the right but can place the hammer head straight in front of themselves already in the first turn.
Throughout 2010 and 2011, Ashe maintained an upright starting position. An introduction in 2012 of a slight forward lean of the trunk on the entry was made. A slight forward lean of the trunk while in DS permitted the thrower (Ashe) to maintain balance when the angular velocity of the body was reduced and the absolute velocity of the implement was relatively high. A thrower of shorter stature like Ashe can take advantage of a longer radius, as it allowed for a smoother change of kinematic indicators.
When making this adjustment, important implications associated with producing a larger radius in the early parts of the throw were considered. For a given linear speed, a larger radius allowed the hammer-thrower system to rotate with a slower angular velocity [45-47]. A slower rate of rotation permitted slower contractions of the muscles involved [45, 46] that allowed these muscles to exert larger forces. This is due to the force-velocity relationship for skeletal muscle [48]. In turn, a larger muscle force results in a larger torque and an increase in the overall angular momentum of the system. Therefore, utilizing a longer radius in the early parts of the throw facilitated an increase in the angular momentum of the system for Ashe [45, 46]. As the trunk straightened at low point in turns two and three and the angular velocity was consequently increased, the rotational radius was gradually reduced.
Ashe successfully added a modest lean to her starting position in 2012 although she originally appeared to be the most comfortable with the upright position. A continuing challenge with Ashe was to transition from the slight forward lean of the first turn to the more upright posture of the second turn. This was one of the technical factors emphasized in 2012 and subsequently in 2013.
The Turns
The smooth transfer from the preliminary swings (winds) into the first turn established the turning rhythm. The motion of the thrower’s center of mass are affected by three forces: gravity, a reaction force exerted by the ground on the thrower’s feet (ground reaction force), and a reaction force equal and opposite to the cable force (cable reaction force) [9,29]. For a good throw, the thrower focused on achieving an appropriate combination of hammer and ground forces that would produce an increase in ball speed [45]. Removal of or lifting the right foot from support was another important training element that was focused on by Ashe. One of the challenges for Ashe was to obtain early right foot placement. To achieve this, her technical training regimen focused on lifting the right leg earlier each turn. The emphasis was on pushing the right foot into the ground until the right foot comes off the ground. This movement was coached to take place when the thrower’s body is at a 90° angle from the frontal plane in the first turn [2].
This strategy permitted the lengthening of the DS phase in relation to the SS phase. Most elite throwers studied attempted to lift the right leg earlier in each subsequent turn as the velocity of the turns increase [25]. However, the degree point of the lift of the right foot was to be reduced to 80° following the first turn and 75° following the second turn [49]. Ashe was instructed to focus on driving the right side so the right leg lift-off occurs more naturally. In theory, the right leg loses contact with the surface of the ring when the angular velocities of the hammer and the athlete’s body are equal and continues until the implement passes its highest point. In order to secure a fast foot placement, the right leg moved in the single-support phase within a minimal radius (coaching cue: tight knees and shins) [49].
Accelerating the Ball
Recently there has been increasing amounts of research into the acceleration mechanism of the hammer [15,50, 51, 52]. The mechanism for increasing or decreasing the radius of rotation involves posture adjustments at the hip and shoulders during the course of the turns [45, 46]. The “Russian Model” served as the basis for this area of technique [12, 25] for instructing the subject. It is also important for athletes and coaches to understand how individual movements of the thrower affect the overall performance. Hammer throw technique in this case study was strongly influenced by movement of the trunk [16, 53]. It is widely accepted that the angle between the shoulders and pelvis (shoulder-pelvis separation angle) increases during SS and decreases during DS as the thrower accelerates the hammer [53-55]. The optimization of the relationship of the shoulders to the pelvis at the initiation of DS was a primary focus of technical intervention utilized in the present case study. The movement of the trunk and shoulders relative to the pelvis has been discussed in coaching literature [25], but has received little research attention.
The build-up and unwinding of the torque that was created between the hip/shoulder axes that produce force was a new concept to Ashe in 2010. The concept was best explained to Ashe using related throwing events (e.g. discus) and sports like softball and golf, which she understood. The optimization of the magnitude of the shoulder-pelvis separation angle has been investigated in a number of other sports that utilize rotations such as discus [56, 57] and golf [58, 59]. From the right foot touch down, the hammer is accelerated to 0 degrees. However, emphasis was given to the optimizing the degrees of acceleration during each turn, for the sake of stability through the overall throw.
The desire was to achieve a modest 20 to 40 degrees of separation during each DS catch phase in favor of a more stable body position [25]. Within each turn, the thrower is to ensure utilization of a technique that results in an increase in hammer speed while also ending the turn in such a position that further increases the speed in the subsequent turn by limiting the deceleration into SS [9,25, 45].
Ashe had a propensity to lead with the head into the next turn (drag the hammer) as she achieved a greater degree of separation. Technically, Ashe was encouraged to reduce the shoulder-pelvis separation angle during the initiation of DS by as much as possible, specifically during turn two. This resulted in a smaller loss in speed during the subsequent SS phase.
Although angles of (shoulder-pelvis) separation in the hammer throw have been discoursed they have not been highly explored in the literature [10,25]. The angle of separation between the shoulders and the hammer is equal to the angle formed from the intersection of the shoulders with an imaginary line from the head of the hammer. The shoulder/hip separation in the DS catch phase, which creates torque that accelerates the hammer, was the key to the hammer thrower’s success in a related study [25]. Proper torque application added to the angular momentum was needed to increase throwing distance [25].
A smooth acceleration pattern proved to be a point of emphasis with Ashe. The emphasis was placed on accelerating the ball by achieving the correct positions during the throw. Countering of the hammer is one characteristic Ashe learned from former American holder Erin Gilreath [25]. In many cases, a disadvantageous hammer radius is created when throwers project forward slightly at the waist in order to increase the radius of the hammer in the early turns by countering with the hips, followed by a countering with the shoulders on turns two and three [53]. As throwers slowly tilt their shoulders and thorax backwards, the radius of rotation shortens which could result in an increase in the linear speed.
The technical challenge for Ashe was the transition from the hip counter in the entry into the shoulder counter in the second turn as an effective shoulder counter is one of the keys to elite hammer performance [25]. The radius shortened when the centripetal forces were great enough that the upper body is engaged in order to help counter the hammer [9]. Maintaining a long radius was possible by the positioning of the head, which allowed Ashe to counter the hammer with her lower body on the initial turn.
In 2010, Ashe occasionally looked ahead of the ball. As a result of turning the head to the left of the implement, Ashe began to bend the right arm which reduced the radius of the rotation and affected balance leading to straightening of the left leg. A technical aspect that was worked on with Ashe was improving her counter to increase her speed in the later turns [25]. When studying her counter the radius decreased in turn 3 in the American junior national (AJR) throw. This technical cue was modeled from the AR (73.87m—242’4”) throw of Erin Gilreath [25] and is not typical for most junior throwers, as inexperienced athletes have difficulty mastering the counter especially to this extreme. In the AJR throw in 2012, Ashe was building up angular momentum during turns one and two and then increasing the angular and linear velocity of the hammer in turn three by decreasing the radius earlier than most. An area that was given considerable attention was the position of the head during the turns. It is generally accepted that looking to the horizon (straight ahead) and slightly upwards helps to maintain the trunk on the rotational axis. Eventually, through repetitious drill sessions, this became a stable part of Ashe’s throw.
The pattern of force development suggests that throwers actively apply force to the hammer as it travels from its highest to lowest points [5,10,60]. By doing this, throwers are also utilizing the effect of gravity whilst actively accelerating the hammer [10,13]. The acceleration pattern of the ball was a technical focus for Ashe. Because she was relatively new to the event, a slower start was emphasized with solid positions and a gradual acceleration. The gradual acceleration is limited to an increase of velocity that is not more than approximately 25% in each subsequent turn. A smooth acceleration of the hammer in the DS phase and gradual shortening of the SS phase makes elite throwers stand out from other throwers. Increasing time in DS and shortening SS are technical skills on which Ashe continues to focus.
Final Delivery
The forces acting on the hammer prior to release include gravity (weight) and the force applied by the thrower to the hammer via the hammer’s cable (cable force) [10,13]. Like hammer velocity, the cable force increases throughout the throw with a single fluctuation occurring within each turn [10,13,49,50,60,61]. Provided the athlete reaches a maximal possible turning velocity and has an effective delivery position with a near-vertical trunk, closely placed feet and a rotational plane of the implement around 40-43°, an effective delivery and long throwing distances are possible even if the athlete demonstrates technical shortcomings in the earlier stages of the throws. As the throw progresses, the decreasing trend of the radius of rotation leads to a reduction in the moment of inertia and an increase in the angular acceleration. Therefore, a shortening of the radius, particularly in the last part of the final turn, could be utilized by throwers to facilitate an increase in hammer speed prior to release [46, 47].
An increased release velocity accounts for the large difference between throw distances from 2010 to 2012. Support phase times showed little information in helping predict throw distance [25]. Therefore, it is thought that other mechanical factors must have contributed to increased performance. The longer times in turns two and three during the 2010 throw indicate a slower rotational speed. Additionally, the radius from the hammer’s center of mass to the axis of rotation must also be considered.
The final delivery action is to take place without a significant backward lean of the trunk (Figure 3). As the implement passes the low point of its trajectory, the legs begin to straighten. As the implement is placed at the level of the athlete’s shoulders, the release of the hammer occurs. Properly executed delivery movements are reflected in a balanced position after the release of the hammer. The biggest difference between Ashe’s release in 2010, 2011 and the AJR throw in 2012, was the balance at release, which was strongly emphasized in training. Countless throws with PUDS, weights, and plates were performed with the emphasis on proper alignment. Many hammer throwers primarily utilize the musculature in the shoulder and arms to generate force. As evidenced by the joint angles in the catch position, the athlete’s hips are in a fairly low, and there is separation between the shoulders and hips, although the upper body is erect [see Figure 3].
As angular momentum is increased, linear velocity of the head of the hammer will also increase.
Ashe demonstrated a very forceful rotary motion in the push-off phase of the throw, but due to a lack of strength, she had trouble maintaining the velocity through the release in 2010 and 2011. A technical adjustment in this area may increase consistency and improvement of the overall throw. The lack of blocking force of the left leg was a possible limiting factor in achieving release velocity. The stopping force of the front leg contributes to the transfer of force to the hammer. In 2012, Ashe accommodated for past deficiencies by improving the power clean and squat 1RM’s. The new strength increased squat and power clean (personal bests improved by 22.6% and 23.5% respectively) combined with a sound technical pattern that sets up the final turn and release, helped to create the velocity necessary to move the implement a record distance.
Strategies to improve technique an increasing velocity were the focus of meetings between the USATF sport scientist, USATF coach’s education coordinator, coach, and athlete. Time spent in DS phase helps to build angular momentum. As angular momentum is increased, linear velocity of the head of the hammer will also increase. Greater increases in angular momentum can be found when engaging trunk rotation along with the torque generated by ground reaction forces [9]. Therefore, focus was given on rotating the lower body around quickly during single support in order for the line through the hip joints to lead the line through the shoulder joints. Additionally, during DS, the trunk rotates back to a neutral position. The meeting discussions determined that good positions were being accomplished, and the main objective of training needed to focus upon improving velocity through conditioning rather than technique modifications.
Recommendations
Throws coaches will benefit from evaluation of this case study as it bridges the gap between the coach and the researcher through integrating biomechanical analysis in teaching the hammer throw. This case study offers guidance to coaches as a background for the analysis of the technique and the planning of the training load. A close relationship exists between technique and performance. Perfecting this technique is a continuous and year-round task. The high level of interest and self-assurance that can be developed within athletes by linking the phase of physics called mechanics to the presentation of fundamental techniques of hammer throwing is likely to amaze both the athlete and the coach. Proof that is based on the evidence of the immutable laws of physics is both captivating and inspiring to athletes [25]. Continuing the development of the understanding of the basic elements of the hammer throw technique (the correct internal image of the technique) is necessary for both coaches and athletes [25]. By adopting the above procedure in the selection and development of fundamental skills, hammer throwers and coaches can have confidence in the soundness of his or her conclusions.
The only way to achieve success in the hammer is by following a consistent training regime that incorporates a system of technical development, overload, progressive resistance, and recovery. Number of throws per year and number of years throwing the hammer, back squat and hammer throwing technique were significant predictors of hammer throw distance in previous studies [25,39,40]. It is important for coaches and athletes to maintain focus. Weight training and lifting programs need to be specific. There is no time to engage in resistance training (i.e. bench press) or other training that is not related to throwing the hammer. Exercises that are to be emphasized include the Squat, and the Olympic lifts. In addition to intensity in the weight room, throws and drills with heavy implements to build “specific throwing strength,” play an integral role in the training regime. Improvements in throwing and general strength will make it possible for the coach to engineer technical advancements. Closer to the season, additional work with lighter implements to build speed, and refine the timing of the technical model is suggested. However, the specific movement pattern of throws with implements of different masses and lengths does not automatically produce the essential changes that are required for enhancement of the competition throws. The projected changes happen only after consideration of the technique of throws with special implements [25].
America’s best female junior hammer thrower, Shelby Ashe, demonstrated a high level of athletic technique. However, despite all the positive elements that are demonstrated by Ashe, the video analysis indicated that there was room for further improvement. To challenge the current world record of 82.98 (272’3”), Ashe must continue to develop key areas physically and technically. Ashe must improve overall explosive strength in order to make improvements in head of the hammer velocity while continuing to focus on the following aspects of technique [25].
1. Improve shoulder counter in the later turns
2. Shorter time in DS vs. SS
3. Early right foot placement and optimum shoulder-hip separation in the later turns
4. Add an additional (fourth) turn to the throw
5. Add an additional (wind) swing to increase initial horizontal velocity
CONCLUSION
Highly effective coaching includes developing a purposeful plan to attack and correct the athlete’s main areas of weakness. Beyond physical characteristics, distinct motor abilities may also help to answer the questions of training emphasis. The coach must create a training regimen that is unique to each athlete, as each will possess unique natural talents as well as specific areas of weakness.
Objective data on the hammer throw can be quantified, measured and studied by researchers and trained coaches. This data can be used to determine the effect of each body segment to the total action. The athlete will benefit from the coach collaborating with a biomechanist to suggest mechanical changes to improve athletic performance. This case study examining the technique and training of American Junior record holder Shelby Ashe bridges the gap between the researcher and the coach through integrating biomechanical analysis as an approach to teaching the hammer throw. This approach engages the utilization of film analysis along with the incorporation of photo sequences as an essential part of the coaching/teaching system. This USATF Coaches education hammer project, which highlights the cooperation between sport science and coaching helped to produce an American junior record of 68.12 meters by Shelby Ashe in the women’s hammer in 2012.
ACKNOWLEDGEMENT
Thank you to the USA Track & Field Coaches Education Committee for their support of this study.
REFERENCES
- Bosen, K. O., A Comparison in the Duration of Acceleration of the Hammer Path in the Single & Double Support Phases, Athletica Asia, 1984, 13, 31-35.
- Bartonietz, K., Barclay, L. and Gathercole, D., Characteristics of Top Performances in the Women’s Hammer Throw: Basics and Technique of the World’s Best Athletes, New Studies in Athletics, 1997, 12(370), 101-109.
- Romanov, I., Women and the Hammer: Some Technical and Kinematic Characteristics, Modern Athlete and Coach, 1998, Oct (36), 35-37.
- Gutierrez‐Davila, M., Soto, V. M. and Rojas, F. J., A Biomechanical Analysis of the Individual Techniques of the Hammer Throw Finalist in the Seville Athletics Worlds Championship 1999, New Studies in Athletics, 2002, 17(2), 15-26.
- Bartonietz, K., Hammer Throwing: Problems and Prospects, Biomechanics in Sport: Performance Improvement and Injury Prevention, 2000, 4, 458-486.
- Burke, E., Pataki, L. and Doherty, K., Sports Performance Series: The Hammer Throw: Fundamental Technique and Strength Plan, National Strength and Conditioning Journal, 1989, 11(4), 4, 8-10, 77-81.
- Judge, L. W. and McGill, K., The Hammer Throw Handbook, Coaches Choice, Monterey, CA, 2014.
- Bondarchuk, A. P., Modern Trends in Hammer Technique, Track and Field Quarterly Review, 1980, 80(1), 39-40.
- Dapena, J., A Kinematic Study of Center of Mass Motions in the Hammer Throw, Journal of Biomechanics, 1986, 19(2), 147-158.
- Brice, S. M., Ness, K. F., Rosemond, D., Lyons, K. and Davis, M., Development and Validation of a Method to Directly Measure the Cable Force During the Hammer Throw, Sports Biomechanics, 2008, 7, 274-287.
- Hay, J. G., The Biomechanics of Sports Techniques, Prentice Hall, Upper Saddle River, New Jersey, 1993, 406.
- Bondarchuk, A. P., The Technique of the Hammer Throw: A Trainer’s Manual for Track and Field, in: Homenkov, L.S., eds., Ultimate Athlete Concepts, Moscow, 1982, 421-437.
- Dapena, J., The Pattern of Hammer Speed During a Hammer Throw and the Influence of Gravity on its Fluctuations. A Three-Dimensional Analysis of Angular Momentum in the Hammer Throw, Journal of Biomechanics, 1984, 17, 553-559.
- Rojas-Ruiz, F. J. and Gutiérrez-Dávila, M., The Relation Between Angular Displacement of the Hammer in the Double Support Phase and its Velocity in the Hammer Throw, Journal of Human Sport and Exercise, 2009, 4, 254-261.
- Maheras, A. V., Reassessing Velocity Generation in Hammer Throwing, New Studies in Athletics, 2009, 24(4), 71-80.
- Morley, M., ‘Hammer Throwing – the Turns Part 1’, The Coach, 2003, 16(May/June), 21-25.
- Knudson, D., Fundamentals of Biomechanics, Plenum Publishers, New York, 2003.
- Kreighbaum and Barthels, Biomechanics: A Qualitative Approach for Studying Human Movement, Benjamin-Cummings Publishing Company, San Francisco, CA, 1996.
- Hunter, I., The Effect of Venue and Wind on the Distance of a Hammer Throw, Research Quarterly in Exercise and Sport, 2005, 76(3), 347-351.
- Plisk, S. S. and Stone, M. H., Periodization Strategies, Strength and Conditioning Journal, 2003, 25(6), 19-37.
- Adams, K., O’Shea, J. P., O’Shea, K. L. and Climstein, M., The Effect of Six Weeks of Squat, Plyometric and Squat-Plyometric Training on Power Production, Journal of Applied Sport Science Research, 1992, 6(1), 36-41.
- Goto, K., Nagasawa, M., Yanagisawa, O., Kizuka, T., Ishii, N. and Takamatsu, K., Muscular Adaptations to Combinations of High- and Low-Intensity Resistance Exercises, Journal of Strength and Conditioning Research, 2004, 18(4), 730-737.
- Bompa, T. O. and Haff, G. G., Periodization: Theory and Methodology of Training, 5th edn., Human Kinetics, Champaign, IL, 2009.
- Stone, M.H., Stone, M.E. and Sands, W.A., Principles and Practice of Resistance Training, Human Kinetics, Champaign, IL, 2007.
- Judge, L.W., Hunter, I. and Gilreath, E., Using Sport Science to Improve Coaching: A Case Study of Erin Gilreath American Record Holder in the Hammer Throw, International Journal of Sports Science and Coaching, 2009, 3(4), 473-484.
- Newton, H., Explosive Lifting for Sports, Human Kinetics, Champaign, IL, 2002.
- Swinton, P.A., Lloyd, R., Keogh, J.W.L., Agouris, I. and Stewart, A.D., A Biomechanical Comparison of the Traditional Squat, Powerlifting Squat, and Box Squat, Journal of Strength and Conditioning Research, 2012, 26(7), 1805-1816.
- Schweigert, D., NDSU Women’s Track and Field Strength Program: Power Events, National Strength and Conditioning Association, 1996, 18(1), 52-60.
- Bencardino, J. T., & Rosenberg, Z. S. Entrapment neuropathies of the shoulder and elbow in the athlete. Clinics in Sports Medicine, 2006, 25(3), 465–487, vi–vii. doi:10.1016/j.csm.2006.03.005
- Neal, S., & Fields, K. B. Peripheral nerve entrapment and injury in the upper extremity. American Family Physician, 2010, 81(2), 147–155.
- Izzi, J., Dennison, D., Noerdlinger, M., Dasilva, M., & Akelman, E. Nerve injuries of the elbow, wrist, and hand in athletes. Clinics in Sports Medicine, 2001, 20(1), 203–217.
- Hayter, C. L., & Giuffre, B. M. Overuse and traumatic injuries of the elbow. Magnetic Resonance Imaging Clinics of North America, 2009, 17(4), 617–638, v. doi:10.1016/j.mric.2009.06.004
- Pagonis, T., Givissis, P., Ditsios, K., Pagonis, A., Petsatodis, G., & Christodoulou, A. The effect of steroid-abuse on anatomic reinsertion of ruptured distal biceps brachii tendon. Injury, 2011, 42(11), 1307–1312. doi:10.1016/j.injury.2011.03.018
- Sahrmann, S. A. Diagnosis and Treatment of Movement Impairment Syndromes, 2001,(1 edition.). St. Louis, Mo: Mosby.
- Sahrmann, S. A. Movement System Impairment Syndromes of the Extremities, Cervical and Thoracic Spines, 2010, (1st ed.). Mosby.
- Cools, A. M., Dewitte, V., Lanszweert, F., Notebaert, D., Roets, A., Soetens, B., … Witvrouw, E. E. Rehabilitation of scapular muscle balance: which exercises to prescribe? The American Journal of Sports Medicine, 2007, 35(10), 1744–1751. doi:10.1177/0363546507303560
- De Mey, K., Danneels, L. A., Cagnie, B., Huyghe, L., Seyns, E., & Cools, A. M.Conscious correction of scapular orientation in overhead athletes performing selected shoulder rehabilitation exercises: the effect on trapezius muscle activation measured by surface electromyography. The Journal of Orthopedic and Sports Physical Therapy, 2013, 43(1), 3–10.
- Magarey, M. E., & Jones, M. A. Dynamic evaluation and early management of altered motor control around the shoulder complex. Manual Therapy, 2003, 8(4), 195–206.
- Judge, L.W., Bellar, D., McAtee, G. & Judge, M., Predictors of Personal Best Performance in the Hammer Throw for U.S. Collegiate Throwers. International Journal of Performance Analysis in Sport, 2010, 10(1), 54-65.
- Judge, L. W., Bellar, D., Turk, M., Judge, M., Gilreath, E. & Smith, J., Relationship of Squat One Repetition Maximum to Weight Throw Performance Among Elite and Collegiate Athletes, International Journal of Performance Analysis in Sport, 2011, 11, 209-219.
- Judge, L.W., Young, M. & Wanless, E. A., Using Sport Science and Training Theory to Develop Elite Performance: A Case Study of a 2005 World Championship Finalist in the Women’s Shot Put, International Journal of Sport Science and Coaching, 2011, 6(3), 365-386.
- Judge, L.W. & Bellar, D., Variables Associated with the Personal Best Performance in the Glide and Spin Shot Put for U.S. Collegiate Throwers, International Journal of Performance Analysis in Sport, 2012, 12, 37-57.
- Judge, L.W., Bellar, D., Thrasher, A., Simon, L., Hindawi, O. S. & Wanless, E., A Pilot Study Exploring the Quadratic Nature of the Relationship of Strength to Performance Among Shot Putters, International Journal of Exercise Science, 2013, 6(2), A-10.
- Reis, V.M. and Ferreira, A.J., The Validity of General and Specific Strength Tests to Predict the Shot Put Performance—A Pilot Study, International Journal of Performance Analysis in Sport, 2003, 3(2), 112-120.
- Dapena, J. and Feltner, M. E., Influence of the Direction of the Cable Force and of the Radius of the Hammer Path on Speed Fluctuations During Hammer Throwing, Journal of Biomechanics, 1989, 22, 565-575.
- Dapena, J. and McDonald, C., A Three-Dimensional Analysis of Angular Momentum in the Hammer Throw, Medicine and Science in Sports & Exercise, 1989, 21(2), 206-220.
- Maroski, R., Optimal Distance from the Implement to the Axis of Rotation in Hammer and Discus Throws, Journal of Biomechanics, 1991, 24(11), 999-1005.
- Hill, A.V., The Maximum Work and Mechanical Efficiency of Human Muscles, and their Most Economical Speed, Journal of Physiology, 1922, 56(1-2), 19-41.
- Petrov, V., Hammer Throw Technique and Drills, Translated Excerpts from Legkaja Atletika, 1980, 8.
- Murofushi, K., Sakurai, S., Umegaki, K. and Kobayashi, K., Development of a System to Measure Radius of Curvature and Speed of Hammer Head During Turns in Hammer Throw, International Journal of Sport and Health Science, 2005, 3, 116-128.
- Murofushi, K., Sakurai, S., Umegaki, K. and Takamatsu, J., Hammer Acceleration Due to Thrower and Hammer Movement Patterns, Sports Biomechanics, 2007, 6, 301-314.
- Ohta, K., Umegaki, K., Murofushi, K. and Luo, Z., Analysis of Hammer Movement Based on a Parametrically Excited Pendulum Model, Procedia Engineering, 2.
- Otto, R., NSA Photosequence 22 – Hammer Throw:’ Yuriy Sedykh 86.74w, Commentary, New Studies in Athletics, 1992, 7(3), 51-65.
- Morriss, C.J. and Bartlett, R.M., Biomechanical Analysis of the Men’s Hammer Throw: Biomechanical Analysis of the 1995 National Championships, in: Bartlett, R.M., ed., British Athletic Federation, 1995, 1, 1-22.
- Morriss, C.J. and Bartlett, R.M., Biomechanical Analysis of the Women’s Hammer Throw: Biomechanical Analysis of the 1995 National Championships, in: Bartlett, R.M., ed., British Athletic Federation, Alsager, 1995, 1, 1-20.
- Leigh, S., Gross, M.T., Li, L. and Yu, B., The Relationship Between Discus Throwing Performance and Combinations of Selected Technical Parameters, Sports Biomechanics, 2008, 7(2), 173-193.
- Leigh, S., Gross, M.T., Li, L. and Yu, B., The Associations of Selected Technical Parameters with Discus Throwing Performance: A Cross-Sectional Study’, Sports Biomechanics, 2007, 6(3), 269-284.
- Chu, Y., Sell, T. C. and Lephart, S. M., The Relationship Between Biomechanical Variables and Driving Performance During the Golf Swing, Journal of Sports Sciences, 2010, 28, 1251-1259.
- Myers, J., Lephart, S., Tsai, Y-S., Sell, T., Smoliga, J. and Jolly, J., The Role of Upper Torso and Pelvis Rotation in Driving Performance during the Golf Swing, Journal of Sports Sciences, 2008, 26(2), 181-188.
- Hwang, I. and Adrian, M., Biomechanical Analysis of Hammer Throwing, in: Adrian, M. & Deutsch, H., ed., Proceedings of the 1984 Olympic Scientific Congress, 1984, 79-86.
- Bartonietz, K., Hinz, L., Lorenz, G. and Lunau, G., The Hammer: The View of the DVfL of the GDR on Talent Selection, Technique and Training of Throwers from Beginner to Top Level Athlete, New Studies in Athletics, 1988, 3(1), 39-56.