This article is adapted from the new book, Running Form: How To Run Faster And Prevent Injury, by Owen Anderson, Ph.D., published by Human Kinetics, Champaign, Il. www.HumanKinetics.com.
By Owen Anderson, PhD
The goals of form improvement include reducing the risk of injury, upgrading performance, and fine-tuning running economy. Another positive, though not automatic, benefit of form improvement is that it often creates a smoother-looking running style. Instead of bumps and jerks due to heel-striking ahead of the body, optimal form involves bouncing rhythmically from foot to foot, giving the appearance of efficiency and grace. It can also feel much more powerful because of the reduction in horizontal braking force during each contact with the ground. The larger fraction of stance devoted to horizontal propulsive force, the more effective use of elastic energy to provide forward propulsion, and the better timing of vertical propulsive force (it will reach a peak at a more optimal time, when the foot is not still in front of the body) are all aspects of good form.
Running Form and Injury Prevention
More than 19 million people finish road races in the United States annually, and around 54 million Americans engage in running at some point over the course of a year (1). Amazingly, 30 million individuals run or jog at least 50 days yearly in the United States, and there are about 540,000 marathon finishers and nearly two million half-marathon finishers annually (2). These encouraging facts are tempered by the realities that approximately 65 percent of regular runners are injured (3) and up to 92 percent of marathon trainees end up on the shelf (4) for some significant period of time during the year.
Science suggests that the use of proper running form can lower these injury rates significantly. From an injury standpoint, the key problems with common form—the kind of heel-strike, foot-ahead-of-the-body form that is adopted by most runners and promotes these remarkably high injury rates—are that it produces higher impact forces with the ground, greater forces at the knee with each impact, increased rates of force loading after impact, and dramatically augmented hip adduction (inward movement of the thigh during stance) compared with optimal form. Optimal form features midfoot-striking with the foot closer to a point under the body’s center of mass. The consequence of the elevated forces and greater hip adduction is a heightened risk of being injured at some point during the training year.
One of the key problems with heel-striking is that it increases an important variable called VALR—the vertical average loading rate of impact force. Research has shown that VALR is the strongest predictor of injury risk in runners; runners with higher values of VALR have greater risks of both bony and soft-tissue injuries (5). In effect, with heel-striking, the impact force experienced by the leg increases too quickly (VALR rises too rapidly), compared with midfoot-striking.
Hitting the ground heel first (as 95 percent of runners do), instead of a midfoot- or forefoot-landing, roughly doubles the risk of running-related injury (6). Heel-striking produces a dramatically higher initial spike in ground-reaction force, compared with forefoot- or midfoot-striking, which is another way of saying that VALR is increased in heel-strikers (Figure 4.1) (7).

As can be seen in figure 4.1, one of the key challenges associated with heel-first collisions is that the loading rate of force applied to the leg upon landing is much higher with heel-striking. In other words, the rate of increase for the force felt by the leg is higher with heel-striking;
the leg experiences the force more rapidly, with less time to react. Higher forces on the leg are linked with a greater risk of injury (8), and higher loading rates of force are also connected with a larger probability of getting hurt (5, 9). Runners who shift from heel-striking to forefoot- or midfoot-landing significantly reduce the magnitude of impact force (10).
How do other aspects of form influence injury rate? Shank angle at initial ground contact (SAT), maximal shank angle (MSA), and Reversal of Sweep (ROS) are relatively new terms in the study of form, so few investigations have been conducted concerning their effects on injury rate. However, SAT and foot-strike pattern are strongly linked. The greater the SAT, the more likely it is that a runner is using a heel-strike ground-contact strategy. Thus, high SAT should be linked with lofty rates of impact-force loading.
The Role of Cadence
Considerable research has explored the link between another related running-form variable, cadence, and the risk of injury. A 2014 study conducted by Rachel Lenhart and her colleagues at the University of Wisconsin at Madison revealed that a 10 percent increase in cadence (step rate) during running reduced peak knee-joint force by 14 percent (11), an effect that should lower the chances of developing patello-femoral pain and knee injury. Previous work by the same group had demonstrated that a mere 5 percent increase in step rate diminished total work performed at the knee per step, reduced the extent of heel-strike at initial ground contact, diminished hip adduction during stance, and also lessened the internal rotation of the hip when the foot was on the ground (12). Stance is the portion of gait during which the foot is in contact with the ground and when it appears that the foot is going backward relative to the rest of the body. In reality, the body is moving forward over the foot, and stance occurs from the instant of initial ground contact to the moment of toe-off. Hip adduction is an inward (medially directed) movement of the thigh during stance. An increased hip adduction angle means that the thigh moves medially and more dramatically during stance, compared with normal hip adduction (figures 4.2 and 4.3).


These factors—reduced peak knee-joint force and lessened hip adduction and rotation—should diminish the risk of knee injury and another important malady called “iliotibial band syndrome” (ITBS). Runners with ITBS generally run with relatively slow cadences (less than 165 steps per minute) and display high degrees of hip adduction and rotation during stance.
While SAT was not measured in the two studies mentioned above, it is important to note that SAT and cadence are strongly linked. The average runner, for example, tends to run with an SAT of about 16 degrees and a cadence of approximately 160 to 164 steps per minute (13). A runner with a cadence of 180 steps per minute, however, often has an SAT of only six to 10 degrees (14). In the studies carried out by Heiderscheit and his colleagues, it is reasonable to assume that as cadence increased, SAT decreased concomitantly, heel-striking was less pronounced; and thus knee-joint forces, hip adduction, and hip rotation were reduced, decreasing the risk of injury.
Common Injuries and Common Form
Research has linked an increased risk of suffering a running-related injury with various form-related body alignment patterns, particularly those patterns displayed by the average runner. Three common running injuries—ITBS, tibial stress fractures, and patellofemoral pain syndrome (characterized by strong discomfort in the front of the knee)—have been linked with increased peak hip adduction angle during the stance phase of gait. The important form-related point here is that hip adduction angle is strongly associated not only with cadence, but also with an important variable called “heel-strike distance” (figure 4.4). This is simply the horizontal distance between the center of the pelvis and the heel at initial contact with the ground: the greater the heel-strike distance, the larger the hip adduction angle (15).
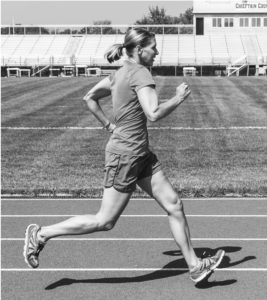
In general, heel-strike distance is a direct function of SAT; the greater the heel-strike distance, the higher the SAT. Thus there is a straightforward connection between SAT and the likelihood of injury. A large SAT leads to large heel-strike distances, which promotes hip adduction and injury.
The increased inward movement of the thigh associated with greater peak hip adduction angle places more stress on the iliotibial band on the lateral side of the thigh and the knee itself and thus can be a source of training-related knee discomfort.
This can be addressed by reducing SAT and thus heel-strike distance. In addition, appropriate, running-specific strength training can improve the strength of the iliotibial band in a functional way and thus reduce peak hip adduction angle, providing runners with further protection from iliotibial band syndrome and knee pain. (Running- specific strengthening techniques are discussed in chapter 14.) Excessive inward movement of the thigh during stance can be considered to be a breakdown in form, which increases the risk of injury.
Overall, an expanded heel-strike distance (and thus larger SAT), increased peak vertical ground reaction force, expanded peak hip adduction, and a decrease in knee flexion at initial ground contact (a straighter leg) have been linked with patellofemoral pain (16) and a heightened risk of both iliotibial band syndrome (17) and tibial stress fracture (18) (figures 4.5 and 4.6).


Exercise scientists recently investigated whether just changing cadence could push these mechanics in the opposite direction, preventing injury. They wanted to determine if increased cadence would lead to shorter heel-strike distance and thus smaller SAT, reduced vertical ground reaction force, better control of the hip during stance, and a more highly flexed knee at impact with the ground (19). It would be rather magical if higher cadence could produce all of these positive results and would certainly reveal how important cadence is for optimal running form.
Cadence Retraining
The cadence research (15) studied heel-strikers who were running about 19 miles a week with a slow cadence, averaging 164 steps per minute. All of the participants went through a six-week “cadence retraining intervention.” To increase cadence, each runner trained (and matched his/her steps) with a metronome, set at a cadence 10 percent greater than the individual’s preferred cadence (in this case, approximately 180 steps per minute). All of the participants completed at least 50 percent of weekly mileage with the cadence uplift of 10 percent (180 steps/minute). They were all guided by either the metronome or another audible tool, such as music with a tempo of 180 beats per minute or a metronome-like smart-phone application.
After six weeks of higher-cadence training, the runners had not raised their natural cadence to 180, but they had improved to about 170 steps per minute. This step-rate upgrade had a number of positive consequences. For example, at 170 steps per minute, the runners experienced a decrease in ankle dorsiflexion at initial ground contact (i.e., they had less-pronounced heel-strikes); their peak hip adduction angle was smaller; and the vertical loading rate of force on the leg was lessened. All of these differences are associated with a lower risk of developing three key running injuries: knee pain, stress fractures, and iliotibial band troubles.
This useful study not only revealed that a simple increase in cadence could produce better running mechanics, but also provided a simple mechanism for improving form for the average runner (by using a metronome set at a 10 percent higher cadence than usual). Using this technique, runners can improve their cadence significantly in a short period of time.
The inquiry also supports other research that has linked increased cadence with changes in gait, and, in turn, the changes in gait are positively linked with a decrease in overuse running injuries (20). Interestingly, the simple act of running barefoot tends to increase cadence and is linked with similar advancements in running mechanics, including a smaller SAT, diminished heel-strike distance, and a reduced reliance on heel-striking (21).
Why does a simple form change such as increased cadence lead to better running mechanics? The quicker cadence tends to bring the foot back closer to the body for initial ground contact. This prevents heel-strike distance and SAT from becoming too large and also shortens the braking phase of stance. Higher cadence also limits the extent of ankle dorsiflexion at initial ground contact (in other words, it minimizes heel-striking). Heel-striking is minimized because at a faster cadence, runners don’t have time to land on the heel, then place the sole of the foot on the ground, and then move through stance to toe-off, as they are unable to maintain a higher cadence with all of this excess action (compared with landing toward the middle to front of the foot and “bouncing” forward).
With a higher cadence and midfoot- to forefoot-striking, the lower limb is placed in a more spring-like landing posture, with a less straight leg, a more highly flexed knee, and a more neutral or slightly plantar-flexed foot at contact with the ground. This allows better distribution of force through the leg after impact and slows down the peak loading rate of impact force through the leg.
When the foot is more closely aligned with the center of mass of the body, the hip tends to be in a more neutral position. It is not as flexed as it is with a large heel-strike distance and a big SAT. Thus, the muscles which control hip adduction (namely the gluteus medius and iliotibial band) have a greater mechanical advantage and are placed in a stronger position from the standpoint of controlling hip adduction. This is another reason why shorter heel-strike distance, smaller SAT, and higher cadence are all linked with better control of the thigh and knee, and thus lower hip adduction and a reduction in impact forces at the knee.
Cadence and SAT are naturally linked together: It is very difficult for a runner to have a high cadence if she has a big SAT. She also can’t have a high cadence if ROS is too minimal because this elongates heel-strike distance and thus the time spent getting the body up and over the foot with each step, increasing the stance phase of gait and automatically slowing cadence. In the study in which runners practiced running with a cadence of 180 steps per minute, the increase in cadence resulted in a decrease in SAT; it is likely this decrease can be attributed to the reduction in heel-strike distance. Conversely, a training intervention that produces a reduced SAT should spontaneously raise cadence. Overall, it is clear that increases in cadence and ROS, and a decrease in SAT, should promote a lower risk of injury. Given the incredibly high injury rate among runners today, it is very important for running athletes to incorporate drills into their training that optimize cadence, ROS, and SAT.
That said, while a shift from heel- to midfoot-striking will certainly reduce stress on the heel, tibia, and knee—and most likely the frequency of heel pain, tibial stress fractures, knee discomfort, and ITBS—it also increases the work that must be performed by the non-heel portion of the foot and Achilles tendon with each step. This temporarily increases the risk of injury to those areas. Therefore, a heel-striking runner should not make a sudden and dramatic plunge into midfoot-landings. Rather, he should very gradually adjust to midfoot-striking over a period of many weeks.
This gradual adjustment can be accomplished by drilling, or using midfoot-strike-enhancing drills on a daily basis. Over the course of many weeks, with daily drill employment, a runner’s “drilling form” and spontaneously adopted running form will gradually approach each other and eventually unify in a way that strengthens the foot, Achilles tendon, and calf and does not produce abrupt increases in force and work output for those regions of the lower appendage.
Running Form and Performance
The best research to date linking changes in running form with actual race performances was carried out by Leena Paavolainen, Heikki Rusko, and others and their scientific team at the Research Institute for Olympic Sports in Jyväskylä, Finland (22, 23). In one investigation, Rusko and colleagues divided experienced endurance runners into two groups. The two collections of athletes were initially similar in ability and trained for the same number of hours (about nine per week over a nine-week period); but the “explosive” group devoted about three of those hours per week to explosive training consisting of short sprints, jumping exercises, hurdling, quick-action leg presses, and high-velocity hamstring curls. In contrast, the control group spent just 15 minutes per week engaged in such activities, instead engaging in larger amounts of traditional endurance training (including steady running at moderate paces).
The control group improved maximal aerobic capacity (VO2max) after nine weeks of training, but they were not able to upgrade 5K performance. In contrast, the explosive group failed to advance VO2max but sped up their 5K times by about 30 seconds (a compromise that most runners would be willing to make).
There were a number of positive changes achieved by the explosive group over the course of the study, including enhanced running economy; heightened maximal speed (measured during an all-out 20-meter sprint); advanced explosiveness during jumping tests; and an upswing in a variable called VMART—the highest velocity attained during a maximal, anaerobic running test on the treadmill. VMART reflects the ability to carry out progressively faster running intervals for a longer time during a challenging workout, or in other words, the development of greater fatigue resistance during high-speed running.
Improvements in running economy and VMART were significantly correlated with better 5K performance. Changes in VMART were strongly linked with decreased contact time, or a shortening of the duration of stance. The stance phase of gait was diminished by about 10 milliseconds per step in the explosive group, which undoubtedly seems small to the casual observer. However, those 10 milliseconds represent extra time “glued to the ground” during running and thus time lost to forward movement. These additional milliseconds add up over the course of a race. To summarize, Rusko’s runners used explosive training to decrease stance duration and thereby increase cadence, a key form and performance variable; therefore, they were able to shave 30 seconds from their 5K performances.
The Three Phases of Stance
It is important to note that the stance phase of gait can be divided into three parts:
1. The braking phase, when the foot has just made contact with the ground and horizontal braking forces are being produced
2. The vertical propulsion phase, when the forces applied to the ground are directed in a vertical direction
3. The horizontal propulsive phase, when the foot’s interaction with the ground produces forward horizontal forces and the runner’s body is propelled forward
Explosive training shortens stance time. Most likely it enhances nervous system responsiveness. This allows a quicker passage through the three components of stance without diminishing horizontal and vertical propulsive forces; it therefore shortens stance in a positive way.
In the second inquiry (24), Rusko and colleagues asked 17 male endurance athletes to take part in a 5K time trial and also undertake a variety of tests of running capacity (including an evaluation of running economy and an all-out 20-meter sprint). They found that 5K performance was significantly associated with 20-meter sprint speed and also with ground-contact time (stance duration) and cadence measured during the 20-meter blast. The higher the cadence and shorter the stance duration during 20-meter sprinting, the faster the 5K.
Foot-strike pattern is also linked with performance. Research has found that faster endurance performers tend to use the midfoot and forefoot ground-contact strategies, while slower runners are more likely to be heel-strikers (25). Anecdotal observations also support the connection between higher-level performance and both midfoot-landing and smaller SAT. For example, elite Kenyan endurance runners tend to run with an SAT of between zero and six degrees, while elite American runners often run with an SAT greater than six degrees. Furthermore, elite Kenyans tend to be midfoot-strikers, while American elites tend to collide with the ground heel first (figure 4.8).
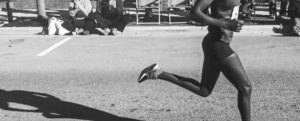
A check of top performances in the world for 2016 reveals that for the 10K (road racing) there were 24 elite male Kenyans in the top 30 and no elite American males. On the women’s side, for the 10K there were 18 elite Kenyans (including two under the author’s management) and only two elite Americans. For the marathon, there were 19 Kenyan men and zero American males in the top 30, and there were eight Kenyan women and zero American females. It is unlikely that the reduced SAT and more pronounced midfoot-landings at touchdown of the Kenyan runners play no role at all in their superiority over American runners. Simply put, great form leads to great performances.
Running Form and Running Economy
Running economy is the oxygen cost of running at a specific speed, and it is strongly linked with endurance performance. In general, the lower the oxygen cost of running at competitive velocities, the faster the performance (26). Just as shorter-duration stance was linked with higher performance in the Rusko research, it is also tightly connected with enhanced running economy (27, 28). Of course, shorter stance time is associated with a smaller SAT and with midfoot-striking, as opposed to heel-striking. These key form variables appear to be directly linked with running economy.
Summary
The running form you choose can either increase or decrease your risk of injury. Deciding to run with a large shank angle at ground contact, a straight leg at ground contact, a heel-strike ground-contact pattern, a relative absence of sweep, or a moderate to low cadence increases the risk of getting hurt.
In contrast, running with an SAT of approximately six to seven degrees, with a flexed knee at initial ground contact, a midfoot- to forefoot-strike pattern, an ROS of about 70 percent of maximal shank angle, and a cadence of 180 or more lowers the chances of injury.
Those factors that advance the risk of injury also harm performance because they are associated with longer stance phases of gait, greater braking forces during stance, and lower cadences. It should be mentioned, too, that injury harms performance by thwarting a key producer of outstanding performance times: consistent training.
Those form factors which decrease the risk of injury are great for performance because they increase cadence, reduce the duration of stance, limit braking effects, and promote the production of maximum propulsive forces during the optimal stage of stance.
References
1. Running USA: Statistics, http://www.runningusa.org/statistics (accessed 2014).
2. Ibid.
3. C.A. Macera et al., “Predicting Lower-Extremity Injuries Among Habitual Runners,” Archives of Internal Medicine 149, no. 11 (1989): 2565–2568.
4. B. Heiderscheit, “Always on the Run,” Journal of Orthopaedic Sports Physical Therapy 44, no. 10 (2014): 724–726.
5. I. Davis et al, “Greater Vertical Impact Loading in Female Runners with Medically Diagnosed Injuries: A Prospective Investigation,” http://bjsm.bmj.com/ (accessed 2016).
6. A.I. Daoud et al., “Foot Strike and Injury Rates in Endurance Runners: A Retrospective Study,” Medicine & Science in Sports and Exercise 44, no. 7 (2012): 1325–1334.
7. Ibid.
8. C.E. Milner et al., “Biomechanical Factors Associated With Tibial Stress Fracture in Female Runners,” Medicine & Science in Sports and Exercise 38 (2006): 323–328.
9. M.B. Schaffler, E.L. Radin, and D.B. Burr, “Mechanical and Morphological Effects of Strain Rate on Fatigue of Compact Bone,” Bone 10, no. 3 (1989): 207–214.
10. Y. Shih, K.L. Lin, and T.Y. Shiang, “Is the Foot Striking Pattern More Important Than Barefoot or Shod Conditions in Running?” Gait Posture 38, no. 3 (2013): 490–494.
11. R.L. Lenhart et al., “Increasing Running Step Rate Reduces Patellofemoral Joint Forces,” Medicine & Science in Sports and Exercise 46, no. 3 (2014): 557–564.
12. B. Heiderscheit et al., “The Effects of Step Rate Manipulation on Joint Mechanics During Running,” Medicine & Science in Sports and Exercise 42, no. 2 (2011): 296–302.
13. Walter Reynolds, personal communication, June 6, 2017.
14. Ibid.
15. J.F. Hafer et al., “The Effect of a Cadence Retraining Protocol on Running Biomechanics and Efficiency: A Pilot Study,” Journal of Sports Sciences 33, no. 7 (2014): 1–8.
16. J.D. Willson and I.S. Davis, “Lower Extremity Mechanics of Females With and Without Patellofemoral Pain Across Activities With Progressively Greater Task Demands,” Clinical Biomechanics 23, no. 2 (2008): 203–211.
17. B. Noehren, I. Davis, and J. Hamill, “Prospective Study of the Biomechanical Factors Associated With Iliotibial Band Syndrome,” Clinical Biomechanics, 27, no. 4 (2007): 366–371.
18. M.B. Pohl et al., “Biomechanical Predictors of Retrospective Tibial Stress Fractures in Runners,” Journal of Biomechanics 41, no. 6 (2008): 1160–1165.
19. J.F. Hafer, “The Effect of a Cadence Retraining Protocol on Running Biomechanics and Efficiency: A Pilot Study,” Journal of Sports Sciences 33, no. 7(2015): 724–731.
20. B.C. Heiderscheit, “Effects of Step Rate Manipulation on Joint Mechanics During Running,” Medicine & Science in Sports and Exercise, 43 no. 2 (2011): 296–302.
21. R. Squadrone, and C. Gallozzi, “Biomechanical and Physiological Comparison of Barefoot and Two Shod Conditions in Experienced Barefoot Runners,” The Journal of Sports Medicine and Physical Fitness 49, no. 1 (2009): 6–13.
22. L. Paavolainen et al., “Explosive-Strength Training Improves 5-km Running Time by Improving Running Economy and Muscle Power,” Journal of Applied Physiology 86, no. 5 (1999): 1527–1533.
23. L.M. Paavolainen, A.T. Nummela, and H.T. Rusko, “Neuromuscular Characteristics and Muscle Power as Determinants of 5-km Running Performance,” Medicine & Science in Sports and Exercise 31, no. 1 (1999): 124–130.
24. Ibid.
25. H. Hasegawa, T. Yamauchi, and W.J. Kraemer, “Foot Strike Patterns of Runners at the 15-km Point During an Elite-Level Half Marathon,” Journal of Strength & Conditioning Research 21, no. 3 (2007): 888–893.
26. K.R. Barnes and A.E. Kilding, “Strategies to Improve Running Economy,” Sports Medicine 45, no. 1 (2015): 37–56.
27. J. Santos-Concejero et al., “Influence of the Biomechanical Variables of the Gait Cycle in Running Economy,” International Journal of Sport Science 36 (2014): 95–108.
28. J. Santos-Concejero et al., “Differences in Ground Contact Time Explain the Less Efficient Running Economy in North African Runners,” Biol. Sport 30 (2013): 181–187.